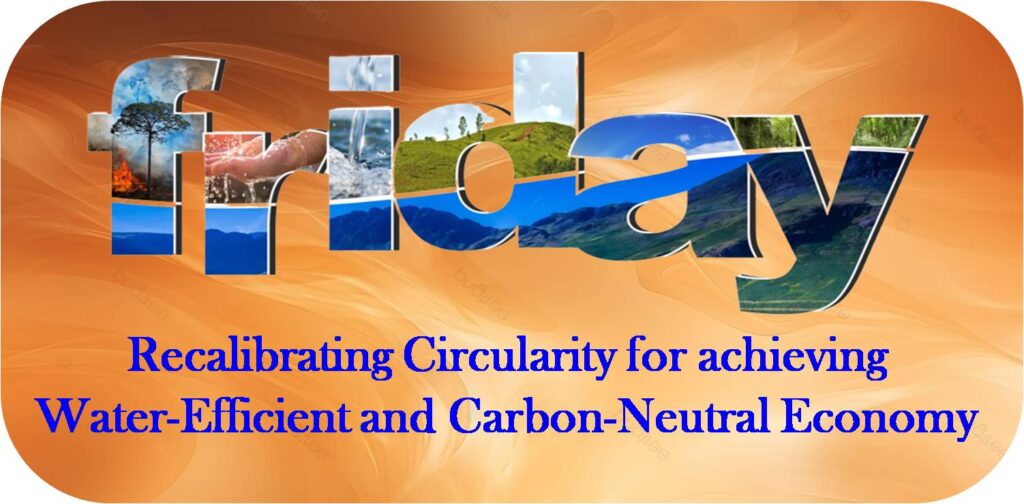
Dr. Arvind Kumar*
The journey to decarbonization and achieving net-zero emissions in the energy sector is critical in mitigating climate change. As part of this endeavor, integrating water circularity provides a strategic pathway to reduce environmental impact while maximizing efficiency. The nexus of energy and water resources highlights the dependency of energy production on water and the need for sustainable water management practices to meet decarbonization targets. Circular water practices reduce the water footprint of energy systems, enhance resilience, and minimize ecological disturbances, paving the way toward a sustainable and low-carbon energy future.The 2024 UNEP Emissions Gap Report highlights the stark choices we face: limit global warming to 1.5°C, struggle to adapt to 2°C, or face catastrophic consequences at 2.6°C and beyond.As per UN as of now, 107 countries, responsible for approximately 82 per cent of global greenhouse gas emissions, had adopted net-zero pledges either in law, in a policy document such as an national climate action plan or a long-term strategy, or in an announcement by a high-level government official.
Water is integral to the energy sector, where it plays a significant role in cooling, processing, and power generation. Thermal power plants, biofuel production, and green hydrogen processes, among others, rely heavily on water resources. However, as climate change threatens water availability and quality, there is an urgent need to shift toward circular water practices. Circular water management aims to reduce, reuse, and recycle water resources, thus decreasing the energy sector’s dependence on fresh water while minimizing wastewater and associated emissions.We need to shift the philosophy of water resources usage from “where to extract clean water and where to get rid of wastewater” to “how to reduce water consumption; how to keep water used in the economy for as long as possible through value retention processes such as rethink, reduce, re-use; and how to regenerate existing water bodies”. The most cost-effective solution is to invest in holistic water management plans that take into account the entire water cycle: from source to distribution, economic use, treatment, recycling, reuse and return to the environment.
As per UNEP a circular economy offers an alternative model, whereby natural resources, including water sources, are kept at their highest economic value for as long as possible.Implementing wastewater recovery and treatment technologies allows energy facilities to reuse water instead of discharging it into the environment. Processes such as advanced oxidation, membrane filtration, and reverse osmosis treat wastewater, making it suitable for reuse in various energy generation processes. For example, treated wastewater can be used in cooling towers and for equipment cleaning, reducing reliance on fresh water sources and lowering pollution levels. By treating and reusing wastewater, the energy sector reduces its water footprint and decreases greenhouse gas emissions associated with water treatment and discharge.
Adoption of Integrated Circular Water Economy
Cooling processes in thermal and nuclear power plants are among the most water-intensive activities in the energy sector. Hybrid cooling systems, which combine wet and dry cooling methods, help reduce water usage while maintaining cooling efficiency. During high-demand periods, dry cooling can be utilized to avoid excessive water consumption, while wet cooling can be used when conditions allow. By reducing water dependency, hybrid cooling aids in minimizing the energy-water nexus’s carbon footprint, aligning with decarbonization goals. Carbon capture, utilization, and storage (CCUS) systems are vital in decarbonizing the energy sector. Integrating water reuse within CCUS processes can reduce water consumption and improve overall efficiency. For instance, water used in carbon capture processes can be treated and reused within the plant for other processes like cooling and cleaning. This approach minimizes water use, reduces emissions, and supports a closed-loop system, contributing to decarbonization and circularity goals simultaneously.
Closed-loop systems involve reusing water within the system, reducing the need for fresh water and wastewater production. These systems capture and treat water from various stages of energy production, enabling reuse in cooling towers, condensers, and cleaning processes. For instance, nuclear and thermal power plants can utilize closed-loop cooling, which significantly reduces water withdrawal. This approach contributes to energy decarbonization by reducing the extraction and treatment of fresh water, lowering emissions, and conserving water resources.
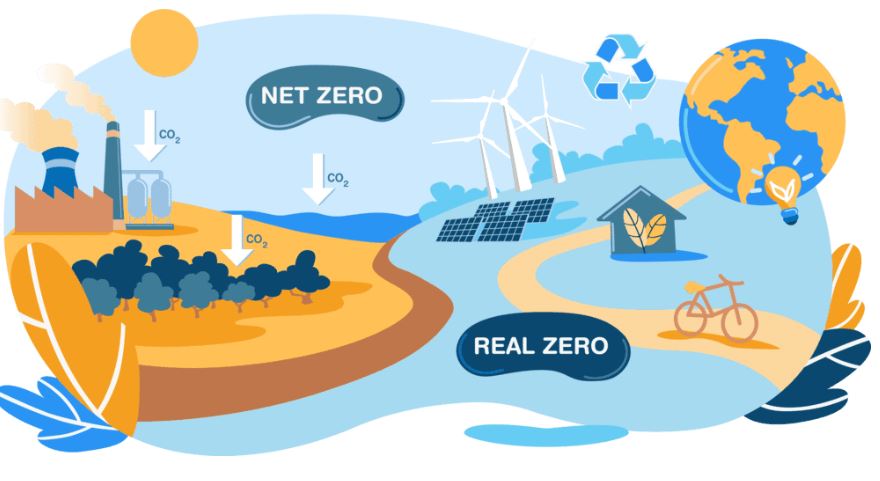
Green hydrogen, produced via electrolysis of water using renewable energy sources, is emerging as a key element in decarbonization strategies. However, hydrogen production requires substantial water inputs. Integrating circular water practices in green hydrogen production involves utilizing recycled or desalinated water, minimizing stress on freshwater resources. Additionally, by coupling hydrogen production facilities with wastewater treatment plants, treated wastewater can serve as the input for electrolysis, conserving freshwater while facilitating green hydrogen production. This integration supports both water and energy circularity and enables a low-carbon energy future.
Renewable energy sources, such as solar or wind, can power water treatment and recycling processes, minimizing the carbon footprint associated with water management in the energy sector. For instance, solar-powered desalination plants convert saline water into usable freshwater, reducing dependency on conventional water sources and providing a sustainable solution for water-intensive energy processes. Similarly, solar-powered electrolysis for hydrogen production lowers the carbon footprint of water use, advancing both water and energy decarbonization. This combination not only saves energy but also reduces greenhouse gas emissions, aiding in the shift to a net-zero energy sector.
Advanced technologies like artificial intelligence (AI), machine learning, and real-time monitoring optimize water usage and enhance efficiency in energy operations. AI algorithms can predict water demand, detect leaks, and suggest water reuse opportunities, thereby reducing waste and emissions. Additionally, real-time monitoring systems track water quality and quantity, enabling proactive water management and minimizing waste. Smart water management reduces energy consumption related to water extraction, treatment, and distribution, contributing to both water and energy circularity.
Singapore has pioneered water circularity through its NEWater program, where wastewater is treated and purified to become a significant source of potable water. This approach has reduced the city-state’s reliance on imported water and freshwater sources. By applying similar techniques in the energy sector, particularly for cooling and hydrogen production, energy facilities could drastically reduce water footprints and emissions.In India, various power plants have started using treated wastewater for cooling purposes, reducing freshwater withdrawals and conserving natural resources. For instance, Maharashtra’s NTPC power plant has implemented a wastewater reuse system, utilizing treated municipal wastewater for cooling and process water, thus contributing to water circularity and reducing the carbon footprint associated with freshwater extraction.California, facing severe droughts, has invested in solar-powered desalination projects to meet water demand sustainably. This initiative provides desalinated water for both municipal and industrial purposes, including energy production. By using solar power for desalination, California achieves water circularity and reduces greenhouse gas emissions, contributing to the overall decarbonization of the energy sector.
By adopting water circularity, energy facilities significantly reduce their reliance on fresh water and the associated energy used for water extraction, treatment, and discharge, thus minimizing their overall carbon footprint. Circular water systems make energy facilities more resilient to water scarcity and climate variability, ensuring a consistent water supply and operational stability. By reducing water usage and reusing treated wastewater, energy plants can lower their operating costs and optimize resource use. Circular water practices reduce wastewater discharge into natural ecosystems, protecting aquatic life and biodiversity.
Way Ahead
Achieving decarbonization and net-zero in the energy sector requires a holistic approach that integrates water circularity. Implementing closed-loop systems, utilizing hybrid cooling, recycling wastewater, leveraging renewable energy for water treatment, and adopting advanced technologies are essential pathways. Despite the benefits, challenges remain in achieving widespread water circularity within the energy sector. High costs associated with advanced water treatment technologies, regulatory constraints, and infrastructure limitations pose barriers. However, advancements in water treatment, increased investment in sustainable infrastructure, and supportive policies can drive progress.Policies should incentivize water reuse, promote investments in renewable energy-powered water treatment technologies, and encourage partnerships between water and energy sectors. By addressing these challenges, the energy sector can move closer to net-zero targets while ensuring sustainable water use.Water circularity not only reduces the sector’s environmental impact but also enhances resilience and efficiency, fostering a sustainable and climate-resilient energy future.
*Editor, Focus Global Reporter